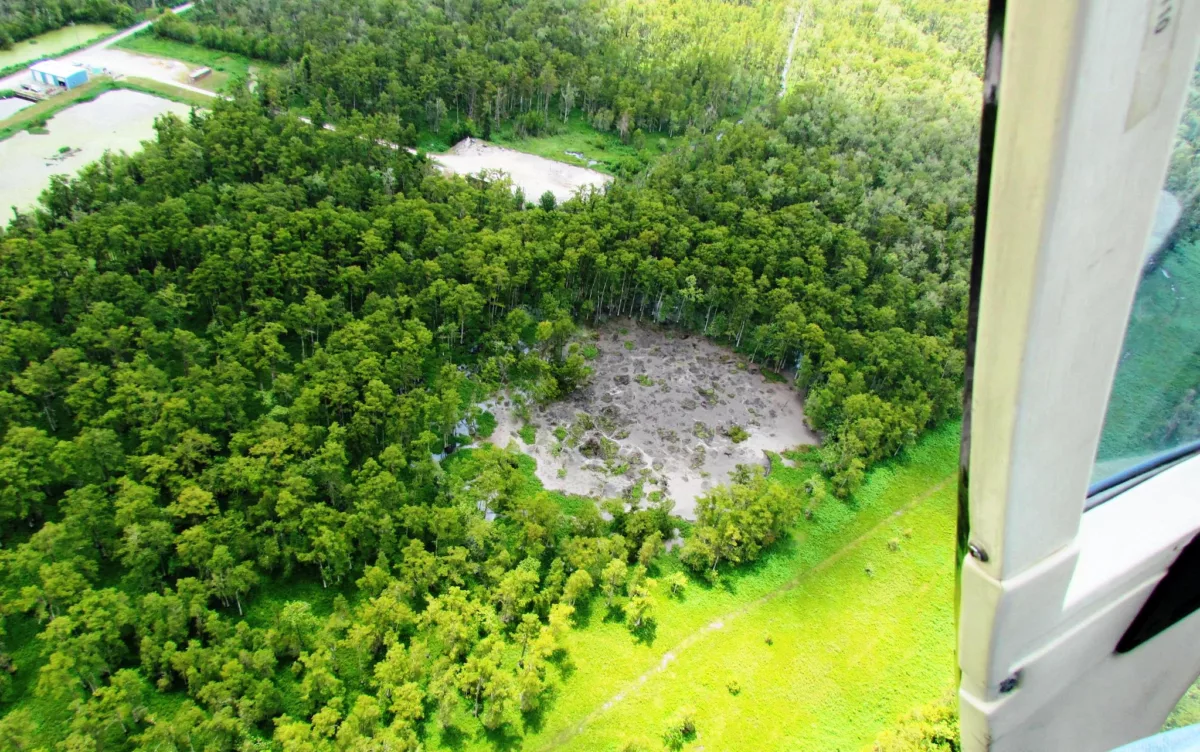
In 2012, a massive sinkhole, along with accompanying gas leaks, forced the evacuation of Bayou Corne, Louisiana. According to news reports, by 2018, the sinkhole had grown to as much as 34 acres. The maw has swallowed cypress trees, and occasionally burped gas that was trapped in shallow sedimentary layers following the accident.
Bayou Corne sits atop the Napoleonville salt dome. Caverns drilled into salt domes like this one provide underground storage for oil and gas. Fields of salt dome caverns, more than 90% of such facilities in the US, are concentrated in Louisiana and Texas. In fact, these two states contain the world’s largest underground supply of emergency crude oil. Salt domes can also be used to store hydrogen, which may play an important role as a future energy source.
Safety for the crews and communities near these facilities is paramount. Mapping structures within salt domes, like shear zones, could help mitigate incidents like Bayou Corne’s sinkhole. Sonar measurements and well logs, obtained by sending instruments down existing boreholes, can provide some insight. However, because they’re expensive and spatially restricted, such data are limited.
Seismic surveys that involve active sources shaking the surface (an example of an active sources is a giant truck called a vibroseis that shakes the ground) have characterized the caprock—the rock above the salt dome—but cannot easily explore deeper structures tucked below, within the salt itself. In a new paper published in Geochemistry, Geophysics, Geosystems, a team of scientists from the University of Arizona and Louisiana State University explored how microearthquakes detected by carefully designed seismic deployments with no active source of seismic waves can provide a way to non-invasively map the internal activities of salt domes.
Salt domes and their shear zones
Salt domes are exactly that—domes of salt that reside underground. Salt’s lower density relative to the rocks above can allow the material to balloon upward. Impermeable rock sitting atop the dome behaves like a seal. This caprock prevents fluids from migrating to the surface. Storing crude oil, natural gas, or hydrogen in salt domes can work well because storage capacity can be large, there’s low requirements for the volume of gas needed to maintain pressure, and salt’s material properties can keep the stored material in place.
However, zones of impurities where surface water or hydrocarbons have infiltrated could create voids called vugs, or cause the salt to recrystallize into friable sections. These zones of weakness may be just that—weak. But, they could also become charged with hydrocarbons, resulting in potentially volatile or explosive conditions, says lead author Joses Omojola, a Ph.D. student at the University of Arizona.
Shear zones around the boundaries of salt domes, appropriately called boundary shear zones, can be meters to kilometers in width, and traverse entire salt domes. If not mapped and avoided prior to excavation of a storage cavern, these zones can feature multiple weaknesses, thereby contributing to failure or leakage of whatever’s stored inside. Between 1959 and 2012, more than 26 incidents, including fires, evacuations, and damage to infrastructure, occurred at salt domes in the US.
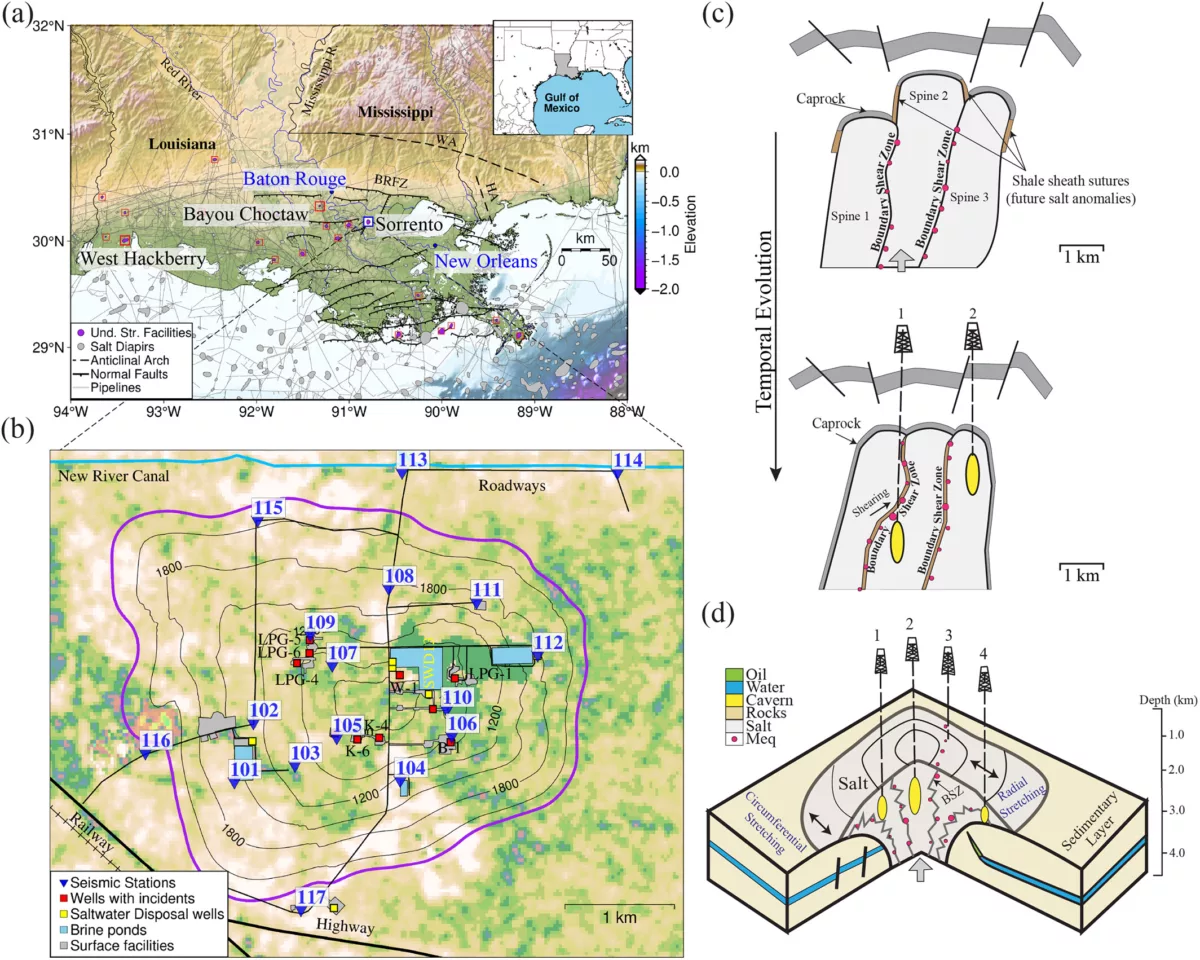
Mapping shear zones via drilling boreholes and seeing what the rock is like directly is an expensive endeavor. Seismic monitoring of small-magnitude events may be more cost-effective, but poses an especially difficult task because small vibrations can be masked by the seismic signature of human-made noise. The rumble of trucks and the whir of machinery can all be detected by seismic stations, making quiet quakes hard to feel. But, there may be a solution.
Tracking Sorrento’s tiny quakes
The Sorrento salt dome, in Ascension Parish, Louisiana, is a 5 kilometer by 4 kilometer diapir that sits below a graben system in which normal faults have offset the central caprock downward. The salt dome is located in the swampy Pontchartrain Basin, a flood-prone region that houses nearly a third of Louisiana’s population. The salt layers here are relatively shallow, with oil and gas production at the north and south flanks. Within the salt dome, underground storage caverns can store 8.92 billion cubic feet of gas.
In January of 2020, cavern operators at the Sorrento salt dome reported ground shaking that seemed unrelated to the typical surficial activities of people. Other incidents resulted in the abandonment of two early wells. And in May of 2021, ground movement damaged a cavern, releasing nitrogen and instigating a 1.5-meter deep crater in the ground beneath the wellhead.
In early 2020, ground shaking reports led Patricia Persaud, a geophysicist now at the University of Arizona, to lead a team, including Louisiana State University students Justin Kain and Ritu Ghose, in the first installation of the 17-station SORRENTO array. With the support of the cavern operators, these installations continued for 12 months spread out over the following two years. Instruments for the early deployments were provided by Rufus Catchings and his group at the U.S. Geological Survey; later deployments used instruments from the NSF SAGE Facility operated by EarthScope.
The team chose nodal seismometers because these instruments can be submerged underwater and do not require solar panels—ideal for regularly inundated swamps. The team buried the seismic instruments in holes about 20 centimeters deep, covering each with soil to reduce noise from highways, equipment, and storms. The stations were deployed as a loosely circular grid spaced about 0.2 to 2 kilometers apart. The team’s goal was to gain insight into the source of the reported ground shaking.
Separating signals
To separate the signal from the noise, the team first developed and trained a model for detecting events and picking phases of salt dome microearthquakes. They used a subset of the Stanford Earthquake Dataset, which is a dataset with earthquakes from all over the world. “We filtered the [database] to select only small magnitude events [less than magnitude 3] at similar event-station distances to what we expect[ed] to record at Sorrento,” Omojola says.
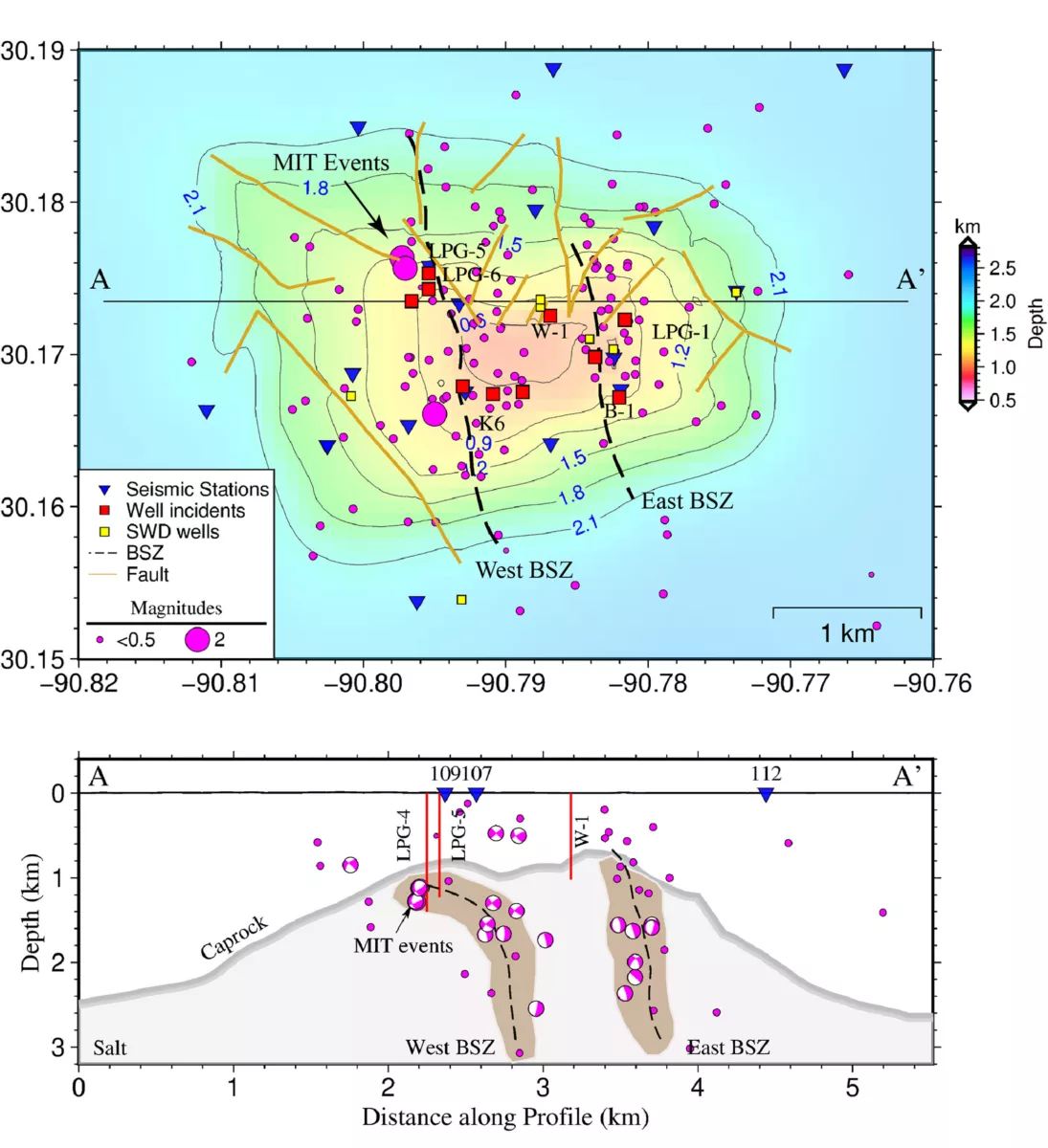
They first trained the model on events from around the world. In this way, the model knew what a general microearthquake looked like. Then, they trained it with 250 manually identified events and 250 random noise waveforms from Sorrento. “This is a useful strategy when you don’t have enough data in your local database,” Omojola explains.
A minimum of four stations was required to detect each event. This helped avoid false positives or spikes of seismicity near individual stations. “Simple actions like jumping beside [seismic stations] could create a spike,” Omojola says. “When looking for earthquakes, we aim to identify signals that arrived at almost the same time across multiple stations.”
Once an event was identified, the team estimated preliminary location and magnitude, and then used additional data to further refine the locations and magnitudes. They were able to divvy up the earthquakes into two batches.
“If the signal was fast and recorded at multiple stations at almost the same time, we called it MS1,” says Omojola. “If it was recorded at multiple stations but at much slower speeds, [we called it] MS2.”
Microearthquakes found
For the MS1 category of quakes, the team found 152 events that exhibited a combination of emergent and impulsive waveforms. A large number of these events originated within the dome at depths between 0.2 and 4 kilometers along two north-south trending zones. Interestingly, they were not aligned with any north-south striking faults above the dome. This pattern suggests that these zones of seismicity are highlighting movement along boundary shear zones—one on the east side and another on the west.
Large-magnitude earthquakes occur almost daily at great distances, yet the microearthquakes would show cascade-like peaks punctuated by days of quiet. This suggests that microseismicity was not triggered by distant quakes. Because few events occurred near the salt-sediment boundary and most struck deep within the dome, the possibility that caprock movements triggered the quakes seems unlikely. Rocks falling from the roof of the cavern could have explained microearthquakes, except that sonar measurements taken every five years indicate that the roof has remained stable while the cavern floor creeps upward.
After eliminating these other possibilities, the authors consider the slow upward migration of salt along shear zones. They see three potential spines of salt migrating upward at different rates, with the central spine rising the fastest. (It also has the thinnest overburden.) “Internal shearing of salt,” they write, “is the dominant source of microearthquake activity at the Sorrento dome.”
Future considerations
The team found far more MS2 events—a total of 1,019. These events were slower and featured impulsive waveforms. “Impulsive means the start of the signal… was not difficult to identify,” explains Omojola. But, the slow wave speeds of MS2 events made them harder to locate. “We know MS2 events were not trains, cars, machinery, or boats because we had verified records of those waveforms, and we could eliminate them as sources,” he says. Nevertheless, their slowness suggests that these were not typical microearthquakes. Swarms of MS2 events coincided with heavy rainfall periods and will be the subject of further investigation.
As more salt domes are used for energy storage, the safety of cavern operations is crucial, according to the authors. Using microseismic monitoring can not only help monitor existing caverns—both operational and abandoned—but also provide information when evaluating new sites.